Notes on Chemical Bonding |
![]() |
When two or more atoms come together it is the rearrangement of electrons that determines whether a chemical is bond is formed and, if so, how strong that bond is and what its properties are. The electrons that are significantly altered when bonds form are the outermost ones. The inner electrons are pretty happy where they are. We find three major types of electron rearrangement. First, there is direct transfer of electrons: some electrons switch from their seats on one atomic ride for the thrill of a ride on another. This type of bonding is called ionic. Second, there is sharing of electrons between two atoms: when the atoms come together they reconfigure their rides so that a seat (or two or three) whiz between the two nuclei. This type of bonding is called covalent (the outer electrons are called "valence" electrons from the Latin word valentia meaning strength or capacity). Third, there is a communal sharing of outer electrons between all the atoms in a metal: the atomic rides are reconfigured so that some of the outermost seats zip from atom to atom, maybe like a bumper car ride in three dimensions. This sort of bonding is called metallic.
The last section described how the periodic table seating chart tells us about the arrangement of electrons in the atoms. We can use it as a guide to the kinds of bonding to expect between different sorts of atoms.
Atoms of the elements on the left side of the table have just a few electrons in the outermost seats. For example, those in the left-most column have one electron in an outer seat. These types of atoms we typically call metals. Theses elements tend not to mind if there outer electron riders are transferred to other atoms, either directly as in ionic bonding or in the communal sharing manner of metallic bonding.
Atoms of the elements on the right side of the table are close to filling all their outermost seats. These elements are called nonmetals. The elements in the right-most column have their outermost seats completely filled and tend not to form strong chemical bonds at all. Those not quite so far right have, with their seats not quite filled like to either pick up a rider from a metal to form an ionic bond or rearrange seats with another nonmetal so that some electrons are riding between nuclei.
This illustration shows an approximate breakdown of metals and nonmetals. The elements in the middle, metalloids, exhibit some metal-like behavior but also form covalent-like bonds. Hydrogen tends to behave more like a nonmetal in bonding except at high pressure at which it actually assumes a phase with the properties of a liquid metal. Since we don't deal with such high pressures normally, hydrogen is often just considered a nonmetal.
The "desire" that an atom of a given element has to acquire a bonding electron rider has been quantified in something called the "electronegativity." While different electronegativity scales have been devised, we'll just use some a typical scale as illustrated in the table below.
The nonmetals tend to have higher electronegativity: they want bonding electrons more than metals. (The "noble gases" are above all this common dealing with electron exchange. Electronegativities for other elements that are white are simply not known yet.) Also notice that, in a given column, electronegativity is higher for elements toward the top of the column. For example, fluorine is the most electronegative element in its column. Chlorine is somewhat less electronegative and bromine, iodine and astatine even more so. You can remember this trend by thinking about the outermost electron riders on the amusement park rides. On the smaller atoms, those toward the top of the table, those outer riders are relatively more important than the outermost riders on the big atoms toward the bottom. For the big atoms with all those electrons already, an extra rider just isn't that important even for elements over on the right side of the table. Astatine, At, in fact doesn't care so much that it behaves as a metalloid rather than a nonmetal.
Let's look at an example of ionic bonding. Lithium fluoride, LiF, is a combination of a metal with a nonmetal, and we would expect ionic bonding. An "ion" is simply an atom or group of bonded atoms that either is missing some electrons (a positive ion) or has some extra electrons (a negative ion). The lithium atom has one outer electron in an s seat. Fluorine has seven outer electrons filling up an s seat and 5 out of 6 spots in the three p seats. The illustration below gives an idea of what the density of electrons around the fluorine and lithium atoms might be.* The darker areas indicate more electron density, that is electrons more electrons spend more time in those regions. The low electron density far out from the lithium center is due to that outer electron in an s seat. The "rings" seen in the illustrations are artifacts of limited resolution when producing the illustrations. The outer electron density seen in the lithium atom is essentially all from the one electron in the outer 2s seat.
Let's imagine a device that can spit out potassium atoms and another that can spit out fluorine atoms. We attach these devices to a metal box and then we attach a pump to vacuum out all the air in the box. We then send in one lithium atom and one fluorine atom headed toward each other. When they get close, the electron in the outer s seat on potassium jumps ship and starts riding on the fluorine atom in the 6th spot of the p seats. Now we have a positively charged potassium ion and a negatively charged chlorine ion, which is often called a "fluoride" ion. The illustration below shows the electron densities after an electron has moved from lithium to fluorine. Note how there is less density far out around lithium and the density around fluorine has increased in size.
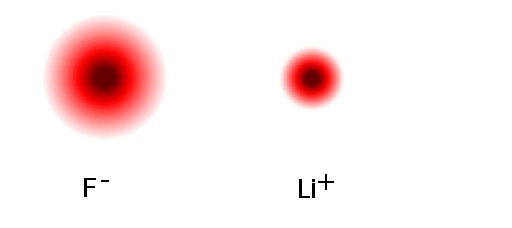
The positive and negative particles formed after the electron transfers attract each other and they come closer. Now, if this pair were in the middle of the box with nothing else around they would smack into each other and bounce apart. Fortunately they were close to the wall of the box and, as a pair, made a collision with the wall so that enough energy was transferred to the wall that the pair could stay bonded together. The illustration below shows what the electron density would look like for a bonded LiF molecule.
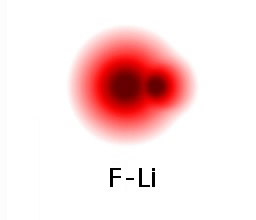
When we find lithium fluoride in its natural state, it exists as a crystalline solid, similar to table salt, NaCl. Many lithium and fluoride ions are arranged in an alternating three-dimensional crystal lattice. It's not possible to really isolate a single Li-F pair in the solid state. The many positive-negative electrical attractions hold the solid together. Ionic compounds are usually solids at room temperature since these electrical attractions are quite strong.
Now let's consider two chlorine atoms, Cl and Cl. Each has a half-empty seat in the out level of sets. But, since both atoms are the same, when the two atoms get close together neither has a greater claim to one of the other's electrons. What they do instead of transferring an electron from one atom to the other, is rearrange the seating. In this case they end up taking the two seats that were half-filled (one from each atom), and make one seat go mostly between the two nuclei and have the other seat fly in some big arc around both nuclei. The seat that goes between the two nuclei is easier to fill and so the two electrons that were in the two atomic seats now both ride in the same "molecular-ride" seat. In this way each atom gets to "share" an electron from the other atom. This sharing turns out to form a fairly strong chemical bond, although typically not as strong as the ionic bonding described above. The atoms in this case also bond just to each other, not to a whole network. This leads to individual molecules of Cl2 in which there is a strong chemical bond. Between chlorine molecules (two Cl2 units) there is not much attraction. Each molecule is an independent ride operator. This helps us understand why, at room temperature, chlorine is a gas. Similar reasoning holds for hydrogen (H2), and in a somewhat more involved manner, for nitrogen (N2) and oxygen (O2). Electron density images for hydrogen are shown in these illustrations.
Finally let's consider two atoms that are different, yet end up sharing electrons. For this case let's consider a hydrogen atom, H, and a fluorine atom, F. Hydrogen acts sort of like a metalloid, so it is somewhere between a metal and a nonmetal. Fluorine is very far toward the non-metallic side. It, in fact, is the most electronegative element, the one most desiring of extra electrons in either covalent or ionic bonding. So, while the bond is not ionic, with hydrogen's electron simply jumping into a fluorine seat, neither is it an even sharing with two shared electrons going between the nuclei. You can probably think of it as a rearrangement of seats, but the seat in which the two shared electrons sit tend to be more like the original seat from the fluorine atom, or at least the electrons spend more time around the fluorine end of the molecule.
Since the electrons are spending a bit more time around the fluorine end, it has a slight negative charge and the hydrogen end is a bit positively charged. The hydrogen fluoride, HF, molecule is a separate entity but the uneven distribution of charge, makes one HF molecule somewhat attractive to another. This results in a group of HF molecules hanging together more strongly the gases described above such as Cl2 and N2. At room temperature, hydrogen fluoride is right on the edge between being a liquid and a gas. At temperatures below 67°F it condenses to a liquid. At 67°F it boils and above that, it's a gas.
*The atomic and molecular electron density images are modeled on figures in "Chemistry from Computers: A New Instrument for the Experimentalist," by Arnold C. Wahl, in Sigma Molecular Orbital Theory by Oktay Sinanoglu and Kenneth B. Wiberg,Yale University Press 1970.
© 2001 bpReid